The research activity at the Energy-Storage Lab joined to the NanoCarbon Lab is focused on the development of innovative technologies for the energy transport, conversion and storage, exploiting novel carbon nanostructures, such as fullerenes, graphene related materials and activated bio-chars. The carbon nanomaterials, which are synthesized and functionalized at the CNL, at the Energy-Storage Lab become components for prototypes of innovative ionic batteries and/or supercapacitors, that are tested to simulate their real use. In this way it is possible to extract important parameters, such as capacity, energy and power density, rate-reliability and life-cycle.
Li-ion batteries
A battery is a device which transforms chemical energy in electric energy, which can be used to power supply electrical devices, such as laptops, smartphone and electric cars. Nowadays, the state-of-the-art among batteries is represented by Li-ion accumulators, which can be charged/discharged many times and which are able to provide the highest energy and power densities.
In a Li-ion battery, Li ions move from the negative electrode (anode) to the positive electrode (cathode) during discharge and back when charging (see the figure below). Usually, electrodes are intercalated lithium compounds, for safety reasons; in particular, the cathode is made of lithium/transition metal (i.e. Co, Mn, Ni…) oxides, while the anode is made of carbon, in form of graphite.
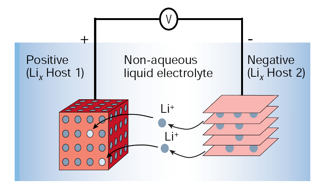
It is worth noting that in Li-ion batteries specific energy (energy per unit of mass or volume) is much lower than common fuels, such as gasoline. Moreover, the scarcity of lithium in nature could pose some limits when the Li-ion technology will be extended on the large scale, such as in electric cars or in the so-called “smart-grids”, for the distribution of energy produced by renewable sources.
However, the development of novel strategies for the electrochemical energy storage exploiting the carbon nanostructures, such as in Li-air batteries or in Li-S batteries, or in novel devices exploiting alternatives to lithium, such as the new Na-ion batteries, is strongly promising for closing the existing gap between batteries and fossil fuels.
Supercapacitors
Supercapacitors, are very promising electrochemical devices that pose between conventional capacitors and rechargeable batteries. Supercapacitors store electrostatic energy by exploiting the charge separation at the interfaces between two porous carbon electrodes and a dielectric (see the figure below).
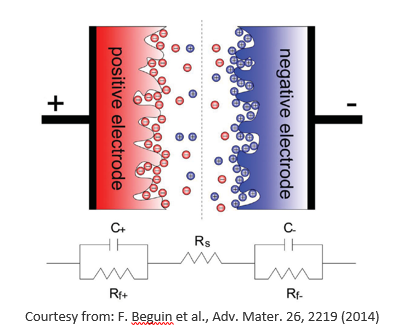
The short distance between the charge layers (few angstroms) and the very high specific surface area (SSA) of electrodes determine much higher capacitance values than those of conventional capacitors, of the order of 10-100 F/g. If compared with rechargeable batteries, supercapacitors show faster charge and discharge rate (higher power density), higher lifetime and lower costs, although their energy density is still much lower than that of Li-ion batteries.
In order to achieve high performance SC, the combination between high SSA with an optimal pore-size distribution of the carbon electrode, suiting the electrolyte ions dimension. is required. Another phenomenon which can boost the supercapacitor performances is the so-called “pseudo-capacity”, namely the possibility to induce faradic (redox) surface reactions on the electrodes, which in that case behave as ultra-thin batteries.
Solid state hydrogen absorbers
Hydrogen is a very efficient energy vector that can deliver three times the energy of the other common chemical fuels. Moreover, when it burns, ti does not produce any greenhouse gases. The main concerns limiting the transition to a “hydrogen economy” are due to the difficulties to develop an efficient hydrogen-storage system, especially for automotive applications. Among the various solution proposed, such as hydrogen compression at very high pressures, or liquefaction at very low temperatures, the best performance is reached by loading hydrogen in solid-state materials, but so far none of them are able to meet the target imposed by industry.
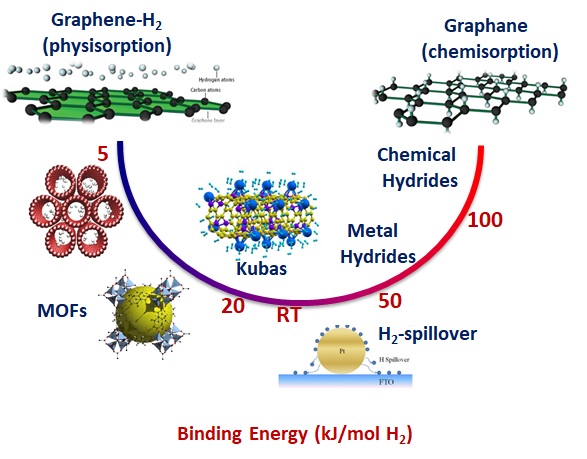
The nanoporous carbon, thanks to the hierarchical microporosity and the excellent mechanical and electronic properties, together with the possibility of decorating it with metal catalysts on a nanometric scale, can allow not only a consistent physisorption of the hydrogen molecules, but also promote new interactions between the hydrogen molecules and the carbon matrix, through for example the so-called “hydrogen spillover” and / or “Kubas interaction”. These mechanisms are known to regulate the hydrogen binding energy to more favorable values than those observed in the processes of physisorption (too weak) and chemisorption (too strong), thus allowing the reversible absorption and release of hydrogen at almost environmental conditions.